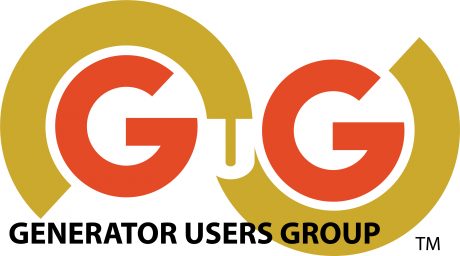
GENERATOR USERS GROUP
GUG: User presentations
GUG: Vendor presentations
GUG: Consultant presentations
Replacement of bushings. The focus of presentations by owner/operators was near equally divided among stators, rotors, and inspection/testing/general. One concerned the replacement of bushings on a nominal 265-MW (1980 COD) hydrogen-cooled generator that runs only four to five months annually at baseload. The well-illustrated presentation covers testing and repairs conducted to enable decision-making, issues faced during replacement (confined space, terminal plate bonded to the bushing plate, etc), and challenges post replacement—including the loss of hydrogen and a blown isophase connection.
Stator, rotor rewinds. The same user also presented on the rewind of both rotor and stator on a 65-MVA, 13.8-kV generator. Periodic maintenance testing revealed a high dc leakage value (120 microamps leakage at 1.25 times the dc value) and a winding resistance unbalance of more than 5%. Plus, rotor inspection revealed shorted turns and loose blocking.
During the rewind, one of the contractor’s staff got a drop of glue in his eye and the use of goggles was made mandatory. After the rewind, the endwinding was re-blocked. Return to service was not smooth, the unit tripping within one second after start attempts. The gremlin: The shorting cable installed on the CT circuit was not removed after generator testing.
Then issues surfaced in the excitation system. Personnel found one diode wired incorrectly on the rotating diode bridge. It took three days to rewire the diode bridge.
EMI testing. A hot topic in generator circles continues to be electromagnetic interference (EMI) testing to detect failing electrical connections—in particular, those inside generators and bus systems. Two significant presentations were made on the topic at the 2019 GUG meeting, with the dialog continuing at the 2020 conference beginning next week.
EMI has been used since the 1950s, possibly earlier, to locate defects in power lines that cause radio and TV interference. Application to powerplant equipment began in about 1980, motivated by the work of several Westinghouse engineers who found that energy discharges in electrical equipment—such as arcing and partial discharge—produce a broadband high-frequency emission pattern. The radio-frequency (RF) currents produced, the engineers said, flow in the machine neutral connection with a magnitude and spectrum signature that can be recognized and differentiated from other RF signals which may be present.
“EMSA (electromagnetic signature analysis) Training,” the first of the EMI presentations last year, is highly recommended by the editors for the background it provides. A significant portion of the presentation is devoted to work done since 2015 by National Instrument and a major utility to develop an affordable instrument for “continuous” monitoring of EMI in generators and transformers.
Fast forward to today, the utility has installed the instrument developed on more than 80 generators and 120 transformers in its fleet. A full-spectrum reading, taken about every 15 minutes, produces data for trending.
The presentation is particularly valuable for what the utility has learned over the years. Here’s a summary of that experience across the four frequency bands of primary interest:
- 30 to 500 kHz. In this region, diode and SCR/Thrystor firing patterns are seen and the following issues can be identified, among others: dirt contamination on diodes, SCRs, and Thyristors; loose excitation cables/connections; missing SCR firing patterns,
- 500 kHz-5 MHz is where issues in the core slots of motor and generator windings likely would be identified—including loose wedges, slot discharge, and back-of-the-core arcing.
- 5 to 30 MHz. Issues associated with motor or generator endwinding structure are likely identified in this frequency band—including loose winding basket, corona in the endwindings, broken strands, etc.
- 30 to 100 MHz. In this frequency band, issues associated with the generator output bus and isolated-phase bus generally are found. Look for cracked insulators, insulator contamination, water intrusion, etc.
Another resource on the subject is the article “Simple EMI Test Detects Failing Joints, Promotes High Plant Availability,” by Consultants Clyde V Maughan and James E Timperley, published in CCJ No. 60 (2019), p 20. You can access it by using the search function on the CCJ home page at www.ccj-online.com
The second presentation last year on electromagnetic interference, “EMSA and IPB,” is a case history spanning several years that probably would be of interest to anyone having had to deal with moisture and other issues associated with isophase bus (IPB). In the end, EMI testing confirmed the generator was not the source of the problem as suspected by most “experts”; rather, it was a defective through bushing from the IPB to the B-phase potential transformer.
Field vibration, thermal sensitivity. One user presented several case histories, each with one or more detailed graphs of meaningful operating data and several highly instructive photos. The first case of an instantaneous increase in generator field vibration was caused by loss of mass. A step change in vibration was noted during a routine startup of the gas-turbine driver. Amplitudes were acceptable to allow the unit to remain in service until a planned shutdown about two days later.
Investigators found a liberated fan blade and stator winding damage when the generator was opened for inspection. The root cause: Improper installation of the bolt locking tab on the affected fan blade.
A second case history presented by the same user also concerned the liberation of a fan blade—this time in the generator coupled to the steam turbine. Once again, the unit was allowed to remain in service following the step change in vibration until a planned shutdown, this time in four hours. Inspection revealed a liberated fan blade and stator winding damage, plus many other bolts well below the design torque value. The root cause was high bending stresses on the bolt which led to fatigue cracking. Note that the fan was designed with a single bolt per blade.
A change in mass distribution caused a step increase in vibration (typically about 2 mils) on yet another generator, but here it was because one of the retaining rings was pushed off-center by the copper winding under certain operating conditions. This has occurred periodically for many years. Correction: Bring the unit to turning gear, then back to speed to reset the retaining ring in the proper position.
Thermally induced vibration attributed to end-turn blocking was experienced by a large 2-pole field with eight coils per pole. Root cause: Blocking between the No. 8 coil and centering ring rotated out of position during installation. At high field current, the vibration increased by 1 to 3 mils and was not consistent in behavior. Multiple compensation balance-shot attempts were made over a five-year run.
Thermally induced vibration attributed to copper binding in slots was the subject of another case history. Investigators found copper yielding in the slot exit area of three coils exceeded the design clearance in the slot. Looking at the generator’s operating history, engineers found the unit had experienced full-load rejection (FLR) a couple of times during the tuning of a new control system.
They believed that over-speed during the FLRs caused the position of the copper to shift relative to existing worn grooves in the slot liners. Copper expansion was limited and vibration increased/decreased proportional to field current. The field was rewound and the affected copper turns replaced.
Accidental energization. Your generator is designed to guard against inadvertent energization, but owner/operators should be aware that unusual conditions that bypass the protection scheme for your machine can occur. And even with protection in place, the speaker said, there is no certainty that an inadvertent energization event will not cause at least minor damage. He noted that energization even for a few cycles is conducive to high current flow and possible arcing.
This introduction to the subject should provide the incentive to read on.
First the background: Accidental energization occurs when 3-phase voltage is suddenly applied to the generator terminals. Such an event is classified as “severe” when the rotor is at standstill or on turning gear.
What happens upon energization is that a rotating flux at synchronous frequency is introduced into the field. High-amplitude currents, similar to negative-sequence currents, circulate in the rotor. The generator behaves like an induction motor and the rotor attempts to accelerate. The result is rapid and excessive heating, particularly at locations of high current density and/or high- resistance connections—such as wedge-to-wedge and retaining-ring interference fits. Damage typically is associated with the rotor forging and rotor components, not the field winding, stator core, and stator winding.
Industry experience, the speaker continued, suggests that inadvertent energization events that are cleared quickly—within one second—will cause minimal damage—possibly no damage at all. However, events longer than a second are almost certain to cause damage, severe damage if the event is more than a few seconds in duration.
The case history presented, supported with many high-resolution photos, describes an inadvertent energization event that occurred when the subject 224-MVA, 20-kV generator (COD 1958) was on turning gear. The machine has a field winding with eight coils per pole and is of the tight-fit wedge design. The field had been rewound in 2003.
The details: A disconnect switch was closed onto a live bus and the stator winding was immediately energized; the normal protection scheme was bypassed. The rotor attempted to accelerate and instrumentation revealed that a speed of 285 rpm was reached. Duration of the fault was 2.1 seconds.
Calculations were made in an attempt to compare the event with values that engineering standards indicate a machine should be able to withstand for a negative sequence event.
Engineers concluded that this event may have exceeded the requirements established by the standards but not necessarily at levels that would suggest severe damage occurred.
Limited visual inspection by experts revealed surface heating, as evidenced by burned paint, had occurred between adjacent slot wedges. Thus, some damage had occurred. Heat-affected metal was expected but couldn’t be assessed without disassembly. Investigators recognized that damage to the forging was possible, but viewed the tight-fit wedge design as having a positive impact.
The consensus conclusion was that the unit could be returned to service provided start/stop cycles were restricted until repairs could be made following a thorough inspection at the next outage in about a year. It appeared that the risk of continued service was not that a catastrophic failure might occur but rather that crack initiation and propagation might worsen the damage and increase the repair scope.
That’s about what happened. Disassembly about 14 months after the event revealed a greater number of burn marks than the optimistic best-case projections based on the partial inspection. Surface discoloration suggested worst-case heat-affected regions were concentrated at wedge-to- wedge interface areas rather than wedge-to-forging areas.
Topics addressed by other user presentations included the following:
- Dc resistance testing provides the capability to detect high-resistance joints, corroded conductors and connections, poor-quality connections, and shorted turns, a highly experienced engineer told the group. Instrumentation capable of very low resistance measurements with sufficient accuracy, precision, and resolution is required and the speaker provided desirablespecifications. Next, the expert outlined a test procedure and reviewed three case studies. Access the presentation for details.
- Endwinding looseness in small machines was the subject of another presentation available in the Power Users library. It covers inspection findings, planning, analysis, repair, and GVPI (global vacuum pressure impregnation) stator ground Lesson learned concerned the need to inspect NGT cable for shielding and grounding.
- Brushless exciter component failure. This is a “how it really happened” case study that begins with the generator protection relay tripping the unit on “loss of field.” The root cause of the problem was found to be a diode module that had failed in such a manner the generator field was shorted out of the The diode modules were rebuilt with new diodes and fuses.
Lesson learned by the presenter was that the sizing of fuses for this application was not so simple as one might expect. In this case, the extended exposure to heat created a condition in which the diode shorted but the fuse did not open.
- In-service failure of an AeroPac II generator attributed to a breach of FME (foreign material exclusion) procedures. No obvious findings were in evidence from an initial visual inspection but electrical testing revealed a grounded phase. The field was removed and metal BBs and other debris was found at the bottom of the frame under the core of the generator in the 6 o’clock A section of the core had melted. A dead-blow hammer was discovered midway in the stator and determined to be the cause of the failure.
The second half of the presentation covered repair options and plan of action. The path taken: The extent of the failure and unknowns in restacking a GVPI unit prompted the owner to swap out the failed generator stator (and rotor) with a spare available in company storage. The equipment, manpower, and schedule required for this effort are detailed in the presentation. FME controls in place at the power producer’s plants were reviewed and modified to avoid a repeat.